(C57 Black/6 mouse) Attacking cancer with the immune system is one of the most enticing dreams of cancer researchers and oncologists. This dream has floated in and out of the foreground for over a century; as early as the 1890s, such a mechanism was probably responsible for a number of “miracle” cancer cures, even if the immune system was still much of a mystery. Since then, the dream of cancer immunotherapy has offered up promising results, but has never fully revealed itself. False summits, but not yet the peak.
The attraction of cancer immunotherapy is easy to understand. While the classical treatments, surgery, radiation, and toxic drugs, are effective, they can be debilitating and often fail to control the subsequent relapse and the metastatic spread of cancer cells to other parts of the body. A mechanism that will hunt down those metastatic cells and destroy them is needed. The immune system is a natural candidate for the job — it’s compatible with the body of the cancer patient, and under the right conditions it can be trained to attack almost anything.
An attractive idea
Several strategies of attack are potentially available, including the use of specifically targeted “killer T cells”. These are lymphocytes that, as part of immune system, can attack and destroy pathological cells, ones infected by viruses or transformed by cancer. One scenario envisages using killer cells that have been isolated from the primary tumour itself. Their presence is evidence that the immune system has responded to the tumour, but its continued growth indicates that the immune system is being stymied in its attempts to destroy it. Enter the cancer immunologists, with their box of tools to manipulate the immune system. One strategy is to help the immune system overcome immunosuppressive effects of the tumour (cancer is the ultimate lethal trickster of diseases); another is to bolster an underpowered immune attack.
A favoured approach to cancer immunotherapy is to collect killer T cells from a tumour and give them a booster treatment outside the body. The emboldened killer cells are then re-introduced near the tumour. Such therapy is called “Adoptive Cancer Therapy”, or ACT. A person whose name has long been associated with ACT is Steven A. Rosenberg, a medical scientist at the National Institutes of Health in Bethesda, Maryland. Rosenberg has been working on ACT, or its precursors, since the late 1980s, in animals and humans (1) (there’s a Wikipedia entry here).
News media like stories about the latest results of cancer immunotherapy. Recently, the New York Times featured a remarkable story about a woman named Celine Ryan. Ryan, who is 50 years old, suffered from advanced colon cancer that had metastasized to her lungs (2) (link to the story here). Thanks to her own strenuous efforts, Ryan was accepted into a clinical ACT trial organized by Dr. Rosenberg. Her recovery, described in December 2016 in the New England Journal of Medicine (3), was little short of sensational. T cells from her tumour were isolated, treated in the laboratory to enhance their tumour-killing activity, and then infused back into her. The tumour enhancement involved detailed analysis of the genes expressed by the killer T cells, and genetically engineering them to enhance their natural anti-tumour activity. She was also given Interleukin 2, a natural growth-stimulating factor for killer T cells. She soon shed the tumour cells that had metastasized to her lungs, and today appears to be in good health (“cured” isn’t a word that oncologists use).
Despite wonderful stories like Ryan’s, cancer immunotherapy is still not generally effective and available, although commercial development has begun (see a recent story here). (Any current applications are going to be very expensive, partly because each patient is a clinical experiment. Their own cells are being analyzed and genetically manipulated.) More work and a better grasp of the mechanism are required.
How ACT was developed and refined
Novel and potentially risky medical procedures need careful development, usually in model animals. Adoptive Cancer Therapy is no exception. Over the decades, countless studies have tried to determine how ACT can be brought to bear on human cancer without endangering the patient. For example, the Rosenberg laboratory continues to fine-tune the protocols in mice (4). Such studies have been going on for decades, in many laboratories, and will probably continue well into the future. The mouse is a good stand-in for the human; it has a similar genome to ours, including the thousands of genes that make up the immune system. Mice have tumours that can be transplanted into other mice for study and manipulation. This is possible because the mice being used are inbred; that is, every mouse in a given strain has the same genes. They are like human identical twins. That means their tissues can be exchanged without risk of rejection (as can also be done with human identical twins). One of the strains used in animal studies by the Rosenberg group is called “C57 Black/6”, abbreviated C57BL/6, or just B6. The reason for the “black” term in the name is apparent from its appearance at the top of this article.
Where did the B6 mouse come from?
After the re-discovery of Mendel’s rules of biological heredity (i.e. genetics) in 1900, some scientists were interested in applying those rules to animals (they were originally discovered in peas, and were also re-discovered in plants). The researcher Dr. Charles Cook Little believed that hereditary factors (genetics) play an extremely important part in determining the incidence of cancer in mice, but also thought that existing systems of study would not be able to clearly establish this (5) (there’s an online version of this reference, here).
What was needed were strains of mice with identical genetic backgrounds. To this end, Little developed a number of inbred mouse lines, including the C57 Black/6 mouse strain. He carried out a series of “back-crosses”, breeding closely-related individuals to each other (“brother-sister matings” — cf. Circei and Jaime Lannister). By doing this, differences in their genotype (the sum of all of their genes) gradually disappeared, until they were for all purposes completely inbred. The B6 mouse was now genetically homogeneous, a growing population of identical twins. The popularity of the B6 mouse with researchers, then and now, is partly related to it being a good breeder and long lived. It tolerates alcohol well, making it a preferred choice for studying the effects of booze on animal physiology. It’s tough, and a little aggressive, and thrives under a variety of circumstances.
The famous English animal geneticist Hans Grüneberg stated that “The introduction of inbred strains into biology is probably comparable in importance with that of the analytical balance in chemistry.” Cancer research, and other branches of animal biology such as immunology, were to become hugely dependent on these little creatures. They are needed in such numbers that a whole industry dedicated to supplying them has grown up.
A mistake leads to a great discovery
Not long after its development, the B6 mouse became part of a mistaken notion about how cancer could be stopped, not the first time there has been a misunderstanding in cancer research. It began with researchers intentionally causing tumours in inbred strains of mice using cancer-causing chemicals (carcinogens). By then, there were a number of such strains in addition to B6. What they soon observed was that a tumour from a mouse of a particular inbred strain could be transplanted into another mouse of the same strain, where it would grow unimpeded. But if the tumour was placed into a different inbred mouse strain, it would shrivel and disappear. Many biologists thought this was evidence of an anti-tumour mechanism, maybe something that could be investigated and developed to treat human cancer patients (by then, the general biological similarity between mice and men was widely accepted by the research community).
The majority opinion on the tumour rejection experiments was wrong. There wasn’t a natural tumour-suppressing substance in mice, at least not a useful one. Instead, what had been revealed was the Major Histocompatibility Complex, MHC, the natural barrier to tissue transplantation. This was teased out by the work of the British scientist Peter Gorer (6). Each of us, like different inbred strains of mice, have molecular markers on the cells of our body. These MHC antigens are our biological identities, and they become targets for the immune system if transplantation of tissue between non-genetically-identical people or mice is attempted. When surgeons transplanted skin from healthy people to burned victims of air warfare during World War II, those transplants were quickly rejected because of MHC differences, unless they came from identical twins (no rejection) or close relatives (slower rejection). Today, organ transplantation between unrelated people is successful only because we have remarkable drugs such as Cyclosporine and Serolimus that block the rejection mechanism.
The existence of MHC differences between people is one reason that physicians such as Steven Rosenberg use cells from the cancer victim to try to pump up the immune response to tumours before putting them back into the patient. If those T cells were from another person, they would be rejected by the patient’s immune system (or they would attack the host cells), and would in any case be ineffective in killing tumour cells.
The mouse was the gateway animal for the discovery of MHC in humans, and a myriad of other discoveries. The B6 mouse played its part in all of this. In 2002, it was chosen to be the first rodent to have its entire genome sequence determined (7). This was a major achievement, similar in difficulty to the determination of the human genome sequence which occurred a little earlier. The genomes of mice and humans are similar in size, just over 3 billion base pairs.
Where did Little get his first B6 mouse?
When C. C. Little was developing the B6 mouse, he was refining a strain that was already at least partly inbred. He obtained his starting mouse from an animal breeder in the little town of Granby, Massachusetts. That breeder was Miss Abbie Lathrop, one of the unsung heroes of biomedical research. Her story is an unusual one.
Miss Lathrop was born in Illinois. She was home schooled for 16 years and then attended a teacher training school for two years. She taught school for a time, but suffered from pernicious anemia, a deficit in red blood cells. There was no effective treatment for her condition, and eventually teaching became too much of an effort. In 1900, at age 32, she moved to Granby, Massachusetts, where her family had roots and property. She decided to create a small business raising chickens, but that didn’t work out, so she changed her business model and began raising pets, including small rodents, for sale to hobbyists.
Among the animals Lathrop began to breed and sell were the strains that were popular with “mouse fanciers”. Just as some people are interested in, and often passionate about, raising and breeding purebred dogs or cats, or orchids, there have been others who care about strains of mice obtained through selective breeding. Compared to dogs or cats, mice cost less to feed and house, and the females are ready to breed six weeks after producing a litter.
Beginning around the 17th century in China and Japan, breeders developed lines of mice by selecting for traits that were easily determined, such as coat colour. Or, in the case of the “pink-eyed Japanese waltzing mouse”, a neurological defect in its inner ear that causes it to spend much of the day spinning around. The “mouse-fancier” hobby spread to Europe, and then America. Descriptive names such as red cream, ruby-eyed yellow, white English sable, and creamy buff, referring to coat or eye colours, could be found in stock catalogues in the nineteenth century. Clubs of mouse fanciers grew up and thrive to this day. Rats are included in some clubs, such as the Rat and Mouse Club of America.
Raising and breeding strains of mice was also a popular hobby in Granby and its surroundings, and Miss Lathrop’s business flourished. In addition to breeding and selling the animals, she showed local children how to care for them, and employed them to help her. She added to the available strains by carrying out her own breeding programs, backcrossing mice derived from wild populations for 12 generations and more.
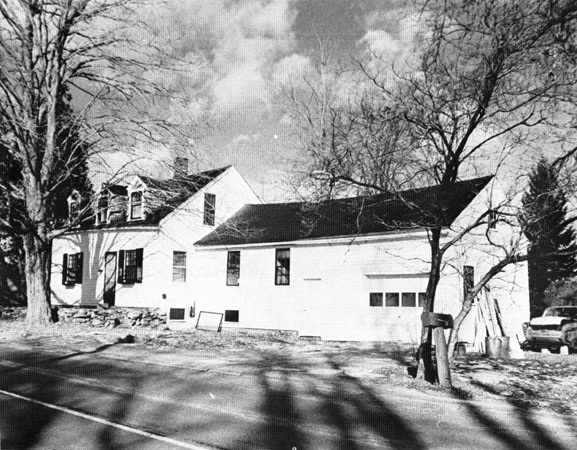
After a few years of selling her mice to hobbyists, Lathrop was puzzled by some very large orders, for hundreds of mice, coming from research institutions. She soon realized that scientists were using mice for research into a number of areas, and scaled up production, and within a few years, her operation was housing more than 10,000 animals. A female mouse identified as “C57” by Miss Lathrop found its way to the laboratory of C. C. Little, and became the progenitor of C57 Black/6.
Miss Lathrop’s cancer-prone mice
Miss Lathrop was a keen observer of the mice in her operation. She kept detailed notes, and some time around 1908, she began to notice that some of her mice were developing skin lesions. She could see that the lesions were occurring at a high frequency in one particular strain, and was interested in what was going on.
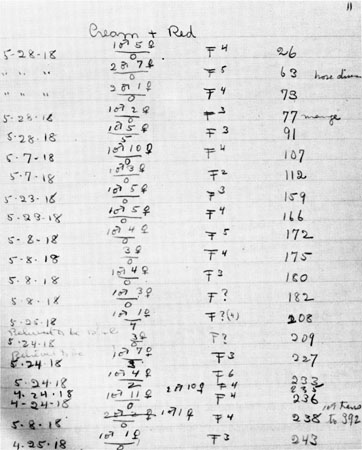
She sent samples of the affected tissues to several research scientists, all but one of whom ignored her request for analysis. She was after all a retired schoolteacher who was raising hobby mice. The exception was Professor Leo Loeb of the University of Pennsylvania, who took the time to examine the tissues sent him by Miss Lathrop, and concluded that the mice had cancer. He contacted her and proposed a series of studies she could carry out. Lathrop and Loeb determined that female mice of some strains had a high likelihood of developing spontaneous mammary tumours. They also found that pregnancy increased the chances of developing mammary tumours, and that removing the ovaries reduced it. They introduced genetics into cancer research, a development dependent on the availability of inbred mouse strains, and which followed from Miss Lathrop’s curiosity about what was going on.
Lathrop and Loeb published a series of 10 papers, the last of them in 1919, the year after she died of pernicious anemia at age 50. Her name appeared first in the bylines of the papers, which were published in some of the most prestigious biomedical journal in America. The first paper was titled “The Incidence of Cancer in Various Strains of Mice”, and appeared in the Proceedings of the Society for Experimental Biology and Medicine in 1913.
It was unusual for a mouse breeder’s name to appear on research publications, then as now. Miss Lathrop wasn’t scientifically trained and didn’t work in a research laboratory. It was an unusual mark of respect and recognition for someone like Professor Loeb to include her name on those papers. She was far more than a breeder of hobby mice; she had a scientist’s keen eye and patience.
The work with mouse tumours by Lathrop and Loeb confirmed Little’s earlier supposition that cancer is a genetic disease, a lesson that was learned, and re-learned over the next 70 years. Inbred mouse strains were instrumental in the discovery of the MHC already alluded to, a fundamental step in the development of transplantation medicine. In biology and medicine, the genes play a role in almost every normal process and disease, and the availability of mice that are genetically identical has been a key contributor to identifying non-genetic components in the environment that influence health and disease.
Because of the growing interest within the biomedical research community, mouse husbandry soon became a serious business. Ten years after Lathrop died, C. C. Little created a large-scale mouse facility at what is today the Jackson Laboratory in Bar Harbor, Maine. This facility continues to be a major supplier and research centre for mouse biology, and ships more B6 mice than any other strain.
Go to Latest Posts
References
- Rosenberg, S. A., B. S. Packard, P. M. Aebersold, D. Solomon, S. L. Topalian, S. T. Toy, P. Simon, et al. 1988. Use of tumor-infiltrating lymphocytes and interleukin-2 in the immunotherapy of patients with metastatic melanoma. A preliminary report. N. Engl. J. Med. 319:1676-1680.
- Grady, D. 2016. 1 Patient, 7 Tumors and 100 Billion Cells Equal 1 Striking Recovery. New York Times December 7, 2016.
- Tran, E., P. F. Robbins, Y.-C. Lu, T. D. Prickett, J. J. Gartner, L. Jia, A. Pasetto, et al. 2016. T-Cell Transfer Therapy Targeting Mutant KRAS in Cancer. New England Journal of Medicine 375:2255-2262.
- Klebanoff, C. A., C. D. Scott, A. J. Leonardi, T. N. Yamamoto, A. C. Cruz, C. Ouyang, M. Ramaswamy, et al. 2016. Memory T cell-driven differentiation of naive cells impairs adoptive immunotherapy. J Clin Invest 126:318-334.
- Morse, H. C. 1978. Introduction. In Origins of Inbred Mice. H. C. Morse, ed. Elsevier.
- Medawar, P. B. 1961. Peter Alfred Gorer. Biographical memoirs of Fellows of the Royal Society 7:95-109.
- MouseGenomeSequencingConsortium. 2002. Initial sequencing and comparative analysis of the mouse genome. Nature 420:520-562.