(Vials of SARS-CoV-2 vaccine).
The coronavirus SARS-CoV-2, the cause of COVID-19, has quickly spread and caused devastation throughout the world. The medical science community has responded vigorously, with better treatments, recommended changes in social behavior, and, of particular importance, effective vaccination programs. Of the immunization protocols that have been developed, the novel mRNA-based vaccines have captured the scientific spotlight; they are the first mRNA vaccines to be licensed for human use.
A new way to immunize
In principle, the mechanism by which mRNA vaccines create immunity to SARS-CoV-2 is straightforward. A version of the mRNA that encodes “S”, the coronavirus “spike protein”, is injected into the body. Some cells take it up and synthesize the S protein, which stimulates the immune system. The resulting response produces immunological protection against infection by SARS-CoV-2. The internet site “How mRNA vaccines from Pfizer and Moderna work, why they’re a breakthrough and why they need to be kept so cold” provides a reader-friendly description of the basics of mRNA vaccines.
Contrary to what is sometimes claimed, often by the vaccine hesitant, mRNA vaccines are not a rushed development; only their recent application to a dire human pandemic is that. The injection of mRNA to reprogram cells in a living organism (a mouse) was successful more than 30 years ago. And the creation of mRNA vaccine is the result of decades of basic research into a wide range of subjects including the biology of bacteriophage (bacterial viruses), chemical synthesis of dinucleotide bonds (the linkages between the nucleotide units of DNA and RNA), and the effects of base substitutions in RNA on the immune system. Among others. It also depended on understanding the complex mechanisms of the immune system itself. But it all came together, with miraculous speed it’s true, in response to the COVID-19 pandemic. Thankfully.
Older conventional vaccines against infectious agents such as viruses use the target molecule (the S protein in this case), produced in cultured cells, to immunize. The Novavax company employs this approach to produce an anti-SARS-CoV-2 vaccine.
A newer type of vaccine is generated with genetically-modified DNA viruses. For the AstraZeneca, Johnson and Johnson, and Gam-COVID anti-SARS-CoV-2 vaccines the viral vector is adenovirus that causes common respiratory infections. The adenovirus is “crippled”, having had sequences necessary for its propagation deleted or destroyed; it cannot replicate. It has been engineered to contain the genetic sequence of the S protein of SARS-CoV-2. The S gene is expressed as an mRNA in infected cells, and this mRNA is translated into the S protein, which stimulates an immune response that blocks infection by SARS-CoV-2.
In principle, mRNA vaccines operate somewhat similarly to those based on adenoviruses. But they differ by directly introducing the mRNA into cells, where it induces the synthesis of the S protein. The vaccinating mRNA is translated into protein for a few days and is then degraded by mechanisms responsible for the turnover of normal cellular mRNA. The S protein is expressed for a relatively brief period, but the immune response to it last a long time (we don’t yet know how long).
The advantages of mRNA vaccines
A useful feature of mRNA vaccines is that they are made entirely by microbiological or biochemical methods, and are relatively easy to purify to human drug quality standards. Conventional protein vaccines, such as the one provided by Novavax, are produced in mammalian cell culture, a more complex medium, from which it is more difficult to purify the vaccine. The same is true of DNA vector vaccines.
The speed at which mRNA can be synthesized, and modified in quantity is one of its most important advantages. The Moderna group produced their first S proteins from synthetic mRNA less than 24 hours after Chinese scientists posted the sequence of SARS-CoV-2. On the other hand, the production of both protein and DNA-based vaccines in large quantities takes longer. The rapid speed of modification and production allows scientists to quickly explore the effects of mRNA sequence variations on efficacy. Basic research on the effects of mRNA sequence on its stability, its inductive properties in the immune system, and its translatability have paved the way for current vaccines.
mRNA vaccines are a significant development in vaccinology, and we were fortunate that the preliminary chapters of this story had been written in the decades before Covid-19 erupted.
The obscure beginnings of a concrete entity
In an otherwise well-written article about mRNA vaccines that appeared in STAT in November, 2020, the writers opined that, “Before messenger RNA was a multibillion-dollar idea, it was a scientific backwater.” I beg to differ. Before Covid-19, mRNA wasn’t heavily featured in the popular press, but it figures in virtually every scientific study and research report in molecular biology. In 2019 there were nearly 26,000 articles in the peer-reviewed biomedical literature alone that mentioned mRNA in the title or abstract. Hardly an obscure entity for the scientists.
But before April 15, 1960, mRNA was not obscure — it was unknown. That was Good Friday, a day of rest in the research labs at Cambridge University. A group of molecular biologists gathered in the college of one of their members to discuss genetic control. By that time, it was known that the expression of genes is regulated: all the cells of an organism contain the same genetic material, but all genes are not expressed at the same time nor to the same degree. Many are expressed in some cells but not in others (muscle does not make insulin, for example). Clever experiments had shown that individual genes can be turned on, or off, quickly. For this to happen there must be a short-lived factor that communicates genetic information from the gene to the protein-synthesizing apparatus of the cell, the ribosomes. Much of the discussion that day centred on, what kind of entity was that factor?
A model for genetic regulation was under construction on the other side of the English Channel, at the Institut Pasteur in Paris. The Cambridge scientists were aware of that work and it figured in their discussions on Good Friday, 1960. One of the scientists designing the model, François Jacob, was in Cambridge that day. The French scientists were studying the ability of some bacteria to “switch on” the metabolism of the sugar lactose when it was added to their growth medium. Absent lactose, the metabolic system for its metabolism was dormant; upon adding it, the necessary genes and proteins were rapidly produced. For this to happen, there must be a quickly-responding messenger to carry the genetic information to the ribosomes. The French scientists labelled it simply ‘Factor X’.
There was a general feeling that ‘X’ was probably RNA, since RNA was a copy of DNA, and there is lots of RNA in ribosomes. In fact, RNA makes up about half of the structure of ribosomes, the protein-translating system, but the structural RNA of ribosomes has none of the desired characteristics of ‘X’; it’s the same in all cells of an organism, and it’s stable. The idea of a messenger RNA, one that quickly changes in response, for example, to lactose added to the growth medium, had appeal. But why had nobody seen it?
François Jacob’s war
The idea of Factor X started on an upper floor of the venerable Institut Pasteur, in Paris, around 1958. Its fathers were the head of the research unit, André Lwoff, and two scientists under his direction, François Jacob and Jacques Monod.
Jacob and Monod each had an interesting World War II. Both were deeply involved in the war effort, Jacob as a medical orderly with de Gaulle’s army of the Free French, Monod as a partisan working covertly to disrupt German operations in France. In June, 1940, Jacob, Like Rick Blaine in ‘Casablanca’, fled through one gate of Paris as the Germans entered another. He and three companions drove south and west, over roads clogged with fleeing civilians. When they reached the Bay of Biscay they snuck on board a Polish troop ship bound for England. There, Jacob enrolled in de Gaulle’s army as an artillery gunner but was quickly re-assigned to a medical unit when it was discovered that he had completed two years of medical school. He served in North Africa for three years, and in June 1944 he landed on the beach during the invasion of Normandy.
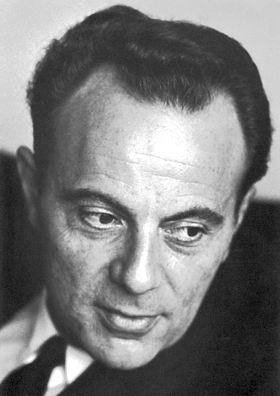
A week after landing, Jacob’s unit was ordered to move out. While refusing to leave the side of an injured man and take cover upon warnings of an incoming bomber attack, he was hit by shrapnel. The injury was serious, and after being transported to Paris, he spent a year in hospital. His arm was permanently damaged, and his dream of becoming a surgeon was finished. Festooned with military honours, Jacob completed the two remaining years of his M. D. degree. He was 25.
The talented Dr. Monod
Jacque Monod was a talented musician and played the cello all his life; at one point, he was torn between pursuing a career in music or in science. Unsatisfied by his biological studies at the Sorbonne, he visited Caltech, where he was exposed to some of the leading figures in the new biology (in California, the good-looking Monod made more inroads into the social high life and musical scene than in science, according to observers). He returned to Paris, completed his thesis, and began research at the Sorbonne. (Upon his return, he received an offer to become the conductor of the Pasadena orchestra; aware of his limitations as an amateur, he wisely declined.)
Then came the War. Jacques Monod had polio as a boy and was lame in one leg as a result. That, together with his age (he was born in 1910) kept him out of the regular army. But by 1940 Monod had joined the Resistance. He also joined the Communist Party, which was necessary for him to have any real influence on the Movement (like many other situational communists, he disavowed the CP when the war ended, enraged by Soviet Russia’s treatment of its scientists). Monod engaged in the kinds of activities described in movies and novels about spies in wartime. At one point, he hid secret papers in the skeleton of a giraffe outside his office. As the War progressed, it became too dangerous for him, as a Jewish communist, to be at the Sorbonne, and he moved continually to avoid not just the Gestapo, but especially the collaborationist French police, who were zealously rounding up 75,000 French Jews for one-way trips to the death camps. André Lwoff, one of the research directors at the Institut Pasteur, offered him space to continue his research there.
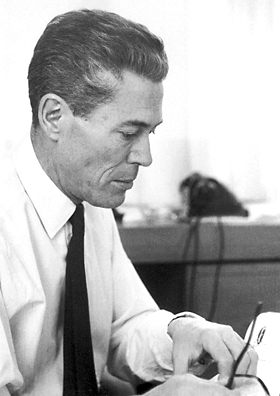
In his clandestine life Monod became Chief of Staff of Operations in the Resistance in Paris and helped coordinate Allied parachute drops of materials and munitions through other parts of France prior to the D-Day landing. After the War, Monod left the Sorbonne for the Institut Pasteur. There, he became interested in the ability of certain bacteria to use the sugar lactose as a food source, and in inducible genetic systems.
Jacob and Monod together
After the experience of the war, François Jacob sought purpose, a meaning in life, but was not motivated in any particular direction. He read widely in science and philosophy, pursued journalism for a short time, tried acting, thought about politics. Then he worked for a while at a pharmaceutical company that was trying to produce the new wonder drug penicillin, but that company failed, and he was adrift again. He had seen a little of research in that job, and was intrigued by it, but felt that he had insufficient background to even attempt it (he was right). Then he met an old friend who had followed the same course of activities during the war and was now pursuing a graduate course in genetics. Jacob felt a surge of enthusiasm — research interested him more than medical practice. Maybe he could do the same as his friend?
To pursue his newest interest, Jacob enrolled in the ‘Great Course’, a comprehensive practical and theoretical course in Microbiology at the Institut Pasteur. One of his lecturers was Jacque Monod; Jacob was impressed and thought that he would like to study under him. To do that he would have to be accepted into the department. The head of the department, Lwoff, turned him down and didn’t encourage him to reapply. But he did, seven times by his own count, and on what Jacob had decided was his final attempt, Lwoff accepted him, to start in 1950. Jacob never knew why Lwoff had changed his mind, and he didn’t care.
Jacob began studying something called viral induction, which refers to bacteriophage which remain dormant in a bacterial genome for a long time, but can then be induced to make virus. Monod was studying the induction of lactose-metabolizing genes. Over time, Jacob and Monod began to see commonalities in their subjects, the inducible systems of lactose metabolism and bacteriophage activation, and began to meld their interests over good coffee and croissants. It was a brilliant insight. A central notion for them was that certain genes could be turned on or off quickly. They began to create an elegant general model for how this happened, some important parts of which, like Factor X, were unknowns at the time.
The conception
And so we come to Cambridge University, April 15, 1960. Jacob and Monod’s model, which had not yet been published, required an evanescent transmitter of information from the genes to the ribosomes. If there was another kind of RNA that accounted for this activity, why had nobody seen it? Suddenly, as recalled by Crick, he, and his colleague Sydney Brenner realized that somebody had seen it. That was the work of Elliot Volkin and Lazarus Astrachan, relative unknowns to the molecular biology big shots gathered that day in Cambridge.
Volkin and Astrachan were at the Oak Ridge National Laboratory in Tennessee, which was created for the Manhattan project. As a result, workers at this lab had access to radioactive isotopes for biological research before other biologists did. Using radioactive precursors of RNA, the ribonucleosides, Volkin and Astrachan observed that after infection by bacteriophage, bacterial cells quickly began to produce a new kind of RNA (1). They didn’t know what this strange RNA was, but it was not a copy of the DNA of the bacterial cells; it resembled the DNA of the bacteriophage. Although they never found out what the new RNA did, their work has been called a “seminal discovery that has never received its proper due” by Nobel laureate Paul Berg of Stanford University.
What suddenly struck Crick and Brenner was that the newly-made RNA seen by Volkin and Astrachan was the messenger. It was synthesized rapidly from the DNA of the infecting bacteriophage, it resembled the genetic content of the bacteriophage, and, it suddenly occured to them, it directed the synthesis of virus proteins. The moment of that realization, Crick wrote in his memoir, ‘What Mad Pursuit’, “. . . was so memorable that I can recall just where Sydney [Brenner], François [Jacob] and I were sitting in the room when it happened.” Like many ideas that became central to molecular biology, it was winningly attractive (Watson had said about the double-stranded DNA model created by him and Crick that it was “too pretty not to be true”). And thus was conceived mRNA, short-lived RNA that transmits information from the genes to the ribosomes.
There was a lively party that night at the home of Francis and Odile Crick, and while the unknowing half of the party-goers had a lively fun evening, the group that had shared the moment of revelation about messenger RNA were plotting how to prove experimentally that their model was correct.
The birth
Jacob and Monod’s monumental paper about gene regulation appeared the next year, 60 years ago this June (2). It outlined how genes can be controlled, and why they must transmit their sequence information to the protein-synthesizing part of the cell through a short-lived intermediate. They proposed three solutions for the nature of this intermediate, their Factor X, but the one they favoured was that it was RNA in form and short-lived in nature: they named it ‘messenger RNA’.
The previous June, two months after the memorable Cambridge gathering, François Jacob from France, Sydney Brenner from Cambridge and Matthew Meselson, a young American scientist, gathered at Caltech. Using a technique pioneered by Meselson, they experimentally demonstrated the existence of mRNA. Their publication, and another paper that reached the same conclusion, were published back-to-back in Nature just two weeks before the magnum opus of Jacob and Monod appeared (3, 4). mRNA was born.
The gestation and birth of mRNA was followed by a period of lavish attention to the exciting infant, and mRNA soon grew to become a mature component of Crick’s famous (and famously misquoted) “Central Dogma”: information travels from nucleic acids to protein, and not in the reverse direction. mRNA is the nucleic acid that carries the genetic information from DNA to the ribosomes. It is indeed Factor X.
The conception, birth, and maturation of mRNA was attended by scientific royalty. Among them, Crick, Brenner, Jacob, Monod and Lwoff won Nobel Prizes. As did a host of other scientists whose work paved the way to mRNA vaccines.
In the past year, after decades of basic research led to the ability to synthesize it in the test tube, mRNA, modified and packaged to ensure its functionality, was injected into millions of human arms. There, it programmed the synthesis of the SARS-CoV-2 spike protein, which stimulated the immune system to make antibodies and cells that neutralize the virus and provide immunity to COVID-19 for millions of people.
Cited sources
- Astrachan, L., and E. Volkin. 1958. Properties of ribonucleic acid turnover in T2-infected Escherichia coli. Biochim Biophys Acta 29:536-544.
- Jacob, F., and J. Monod. 1961. Genetic regulatory mechanisms in the synthesis of proteins. Journal of Molecular Biology 3:318-356.
- Brenner, S., F. Jacob, and M. Meselson. 1961. An Unstable Intermediate Carrying Informatiion From Genes to Ribosomes for Protein Synthesis. Nature 190:576-581.
- Gros, F., H. Hiatt, W. Gilbert, C. G. Kurland, R. W. Risebrough, and J. D. Watson. 1961. Unstable ribonucleic acid revealed by pulse labelling of Escherichia coli. Nature 190:581-585.
Go to Latest Posts