A death in Minnesota
The young man arrived in the emergency room in Minneapolis, Minnesota in acute distress, with a fever, rapid deep breathing, and a racing heart. As reported in 2018, doctors couldn’t initially identify the cause of his problem (1). His breath had twice the normal level of carbon dioxide, and that level kept going up. His fever also kept increasing, despite the doctors’ frantic efforts to stabilize him. Forty-five minutes after admission the muscles in his body violently seized up, rigor mortis, but in a body not yet dead. The rigidity was so hard, the tube the doctors had inserted into his trachea to help him breathe was clamped off, and no amount of physical effort could unblock it. Fifteen minutes after that, the CO2 in his breath reached three times normal. All attempts to save him failed, and ninety minutes after the young man reached the hospital he died. His temperature at the time of death had soared to 43.1ºC (109.6ºF).
Previous to the medical incident, the patient was a healthy 26-year-old. But twelve hours before his appearance in the emergency room he had taken an illicit drug called dinitrophenol (DNP), which he had purchased from a website. DNP was used in munitions manufacture in World War I, and then as a pesticide, a dye, and a wood preservative. In 1933 it was discovered that DNP led to rapid weight loss, and for a few years after that it was marketed as a safe, over the counter, weight loss drug. It was even recommended by physicians (2). However, some nasty side effects began to occur, and in a few cases people died. There was only a narrow range of concentrations in which it led to safe weight loss. By the end of the 1930s, it was no longer being prescribed by doctors, and was taken off the market. There were only a few deaths after that, some of which were intentional — it was occasionally used as a suicide agent.
In the twenty-first century, DNP became available on the Internet, and its use began to increase again. It is an effective weight-loss agent, and some bodybuilders use it to help provide “definition” to their bodies. As its use increased, deaths also began to occur: between 2012 and the middle of 2018, there were 23 deaths from DNP toxicity in England alone (3). Although it is illegal to market it for human consumption in North America, it can be purchased online from European sources on eBay. The young man in Minnesota had purchased it as a “Shrub and Plant Fertilizer” online, where it is marketed as a fertilizer, but often sold in capsule form, obviously for human consumption.
Similar symptoms, including uncontrolled hyperthermia (high fever) and tachycardia (very fast heart rate) have been seen after poisoning by certain other agents. One of these is pentachlorophenol (PCP), which is a wood preservative and a disinfectant (it is unrelated to the hallucinogenic drug phencyclidine, angel dust, which is also abbreviated PCP). Because of its toxicity, the countries that signed the Stockholm Convention in 2015 voted overwhelmingly to ban the use of pentachlorophenol. Its toxicity is illustrated by a report from the late 1960s in which nine newborn babies in an American hospital developed high fever, a racing heart rate, and respiratory distress. Seven recovered, but two died. They had been poisoned by PCP used to launder their diapers and bed linen (4). Other incidences of PCP poisoning have been reported, usually the results of contact with furniture or construction wood that had been treated with PCP as a preservative.
DNP and PCP poison in the same way: they destroy the integrity of the body’s fundamental energy-producing system, short-circuiting the potential energy that drives all cellular function, and turning it into heat that causes a raging fever. The phenomenon responsible is called “uncoupling”, and it goes to the heart of animal life. To understand it requires a side-trip into cellular energetics. WARNING: IT GETS A LITTLE WONKISH.
Energy metabolism
A burning candle produces heat and light as it converts wax to carbon dioxide. Cellular energy metabolism converts the molecules derived from dietary carbohydrate, protein, and fat to CO2. Both the burning candle and energy metabolism produce CO2 and use oxygen, but there are big differences. For one, energy metabolism operates at body temperature. It does produce heat, but the heat is removed from the cell, and keeps the body warm. If excessive heat is produced, it is shunted to blood vessels in the skin, and we sweat. While the carbon of the candle wax combines directly with oxygen at high temperature, energy metabolism proceeds by a series of discrete, enzyme-catalyzed reactions of far greater complexity. The objective of energy metabolism is to produce adenosine triphosphate, ATP, the molecules that is sometimes referred to as the energy currency of life.
ATP is the source of energy for muscle contraction, ion transport, protein synthesis . . . almost all of the energy-requiring processes of the body. Those processes utilize the chemical energy of ATP by breaking it down to adenosine diphosphate, ADP, and inorganic phosphate, Pi:
ATP ——> ADP + Pi
The structures of these intermediates are:
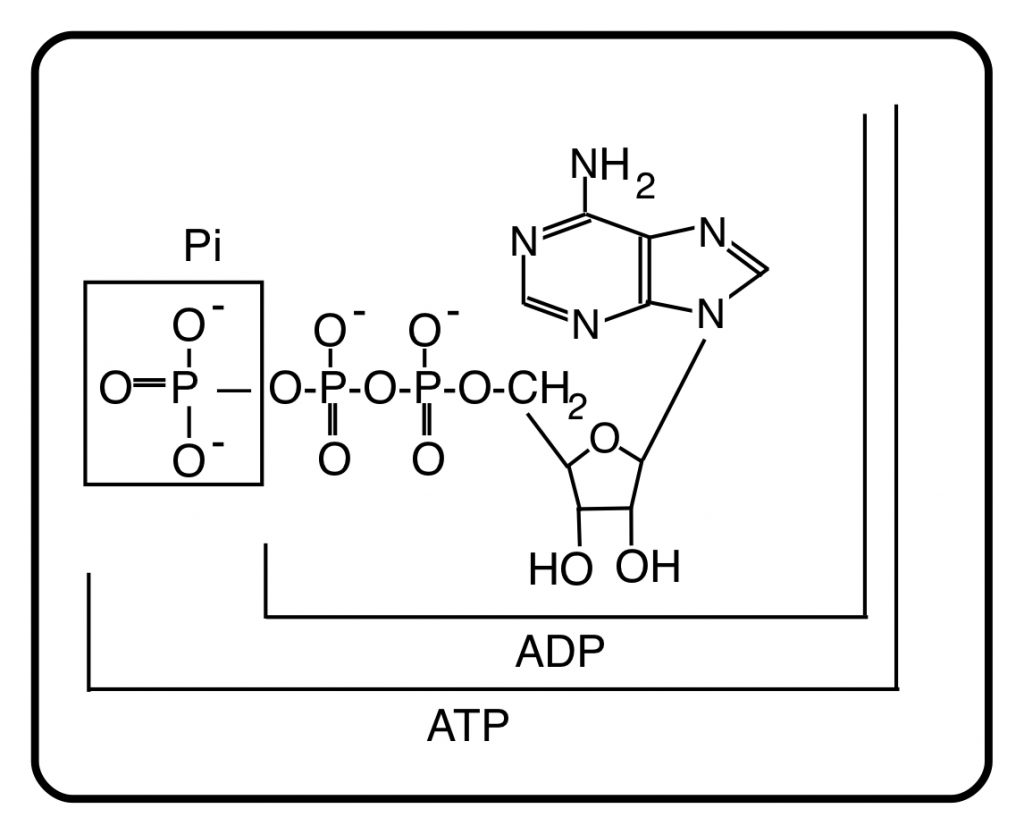
The primary function of energy metabolism is the resynthesis of ATP from ADP and Pi. The oxidation of substrates that are the breakdown products of our food drives this resynthesis, which is dependent on oxygen. The metabolic scheme that drives at least 90% of ATP resynthesis is “oxidative phosphorylation”, a cumbersome name, but also an accurate one. It is the main mechanism that re-phosphorylates ADP to ATP using oxygen.
Peter Mitchell’s vision
Discovering the biochemical mechanisms and molecules of cellular metabolism was a triumph of twentieth century science. The intermediates, enzymes, and control mechanisms of the myriad pathways were determined by studies in a host of organisms, which share most characteristics of metabolism as a result of evolution from common ancestors. As of 1960, however, there was a blank space where the mechanism of oxidative phosphorylation should be. Conventional biochemistry did not yield a convincing description of it, even after its overall purpose and function were well understood.
I can remember, as a graduate student in the 1960s, senior scientists often asking each other variations of the question “Do you believe in the Mitchell Hypothesis?” The question referred to a radical model for how oxidative phosphorylation converted the energy produced by oxidizing substrates to CO2 to generate ATP. The creator of this strange new idea was Dr. Peter Mitchell.
Peter Mitchell, who died in 1992, was almost as unusual as his hypothesis about oxidative phosphorylation. Most notable scientists are good, even excellent students in their younger years. Mitchell was not. He was good at Mathematics and Physics, but not in more descriptive subjects — he wanted to understand things from first principles; memorization did not appeal to him. He failed the scholarship entry exam to Cambridge University, but was admitted anyway on a personal recommendation. At university, he performed at only a mediocre level in his first two years, and slightly better in his third (undergraduate programs at Cambridge were three years long). But he must have impressed the faculty, including its first Chair, the internationally renowned Frederick Gowland Hopkins. Gowland Hopkins obviously saw some research potential in this middling student, and he was taken on as a graduate student in the department despite his mediocre undergraduate grades.
Even as a graduate student, Mitchell began to think about cellular chemical reactions in different terms than most biochemists did. Then, as now, most biochemists thought of chemical reactions in living systems as occurring in a homogeneous solution. The term is “scalar reactions”. But a cell, as has famously been said, is not a bag of enzymes. In the first place, the interior of cells is not a thin watery soup: it is viscous and thick with molecules, and those associated with a particular function often cluster together. Even more definitively, cells are compartmentalized, and different functions happen in different compartments, or organelles. The nucleus, where DNA synthesis takes place, is one of these. Protein synthesis mostly happens outside the nucleus, in the “extranuclear” compartment. Other organelles degrade and digest molecules destined for destruction.
Mitchell was interested in biochemical reactions that are “vectorial”. Vectorial reactions, as distinct from scalar ones, have a physical directionality. For example, a protein that transports sodium across the cell membrane has a direction; it pumps sodium from inside to outside the cell. Mitchell saw that kind of process in other phenomena as well, and wanted to look for and characterize it further. But first, he finished what he thought was a novel and provocative PhD thesis. It was rejected; one examiner described it as inchoate and incoherent. His struggle to become a self-directed scientist continued.
Mitchell re-worked his thesis. It was accepted, and he moved on to an independent research appointment. A few years after that, in 1955, he obtained a position at the University of Edinburgh. There, he began to develop his ideas about vectorial metabolism, in particular to how it might apply to oxidative phosphorylation. He first presented his work publicly in 1961, and got a very sceptical reception (5). He continued to develop his ideas. In 1963, he left Edinburgh because of severe ulcers. For most scientists, that might have spelled the end of a research career, but Mitchell held an ace and he had a dream: he was receiving a trust income from the Wimpy Construction company, which had been started by an uncle. This allowed him to follow his dream.
To realize their dream, he and his wife remodeled a historically important mansion in Cornwall, Glynn House, as a home and a research laboratory they named the Glynn Research Institute. They established a charitable organization to fund his work on bioenergetics. But even with his endowment, Mitchell could only support a three-person research operation. But it was this small, focused group that brought to life Mitchell’s “chemiosmotic” hypothesis, describing how the energy produced by the oxidation of food was captured as the bond energy of ATP.
The Mighty Mitochondrion
The nexus of Peter Mitchell’s hypothesis and the death in Minneapolis from DNP toxicity is the mitochondrion. Mitochondria (cells typically have many) are organelles that carry out a number of functions, including setting off cellular suicide when the cell becomes too damaged to be fixed. But their everyday activity, for which they are exquisitely designed, is to take in substrates derived from food, and to regenerate ATP from ADP through their oxidation. Evolutioary theory proposes that mitochondria began as a bacterial cell that was captured (engulfed) by another microbial cell called archae. The genetic constitutuents of mitochondria are consistent with this idea.
When Mitchell started growing his hypothesis, it was already well established that mitochondria were the cellular site of oxygen-dependent ATP synthesis. For example, rat liver mitochondria, incubated in a test tube, use substrate molecules to drive the synthesis of ATP from ADP by an oxygen-dependent mechanism. It was the mechanism that was baffling. Thousands of person-years of research hadn’t provided a chemical description of it, and one by one, proposed mechanisms based on solution chemistry, scalar reactions, were discarded.
Mitochondria have a unique architecture. Like other organelles, they have an outer membrane to keep their contents from leaking into the rest of the cell. But there is also a second, highly invaginated inner membrane. This was the part of the structure that Peter Mitchell focused on. He was familiar with vectorial (directional) cellular processes, for example the transport of sodium out of cells. Such transport is often against a concentration gradient (there’s more sodium outside the cell than inside it), and requires energy, which is often provided by ATP. Could the reverse process, allowing ions to flow down a concentration gradient, produce ATP? I’ve read, with difficulty, how he came to formulate his model for oxidative phosphorylation, and I still don’t know whether he was thinking along those lines, but it’s a logical connection. What he did think was that vectorial metabolism across the inner mitochondrial membrane was the basis of oxidative phosphorylation.
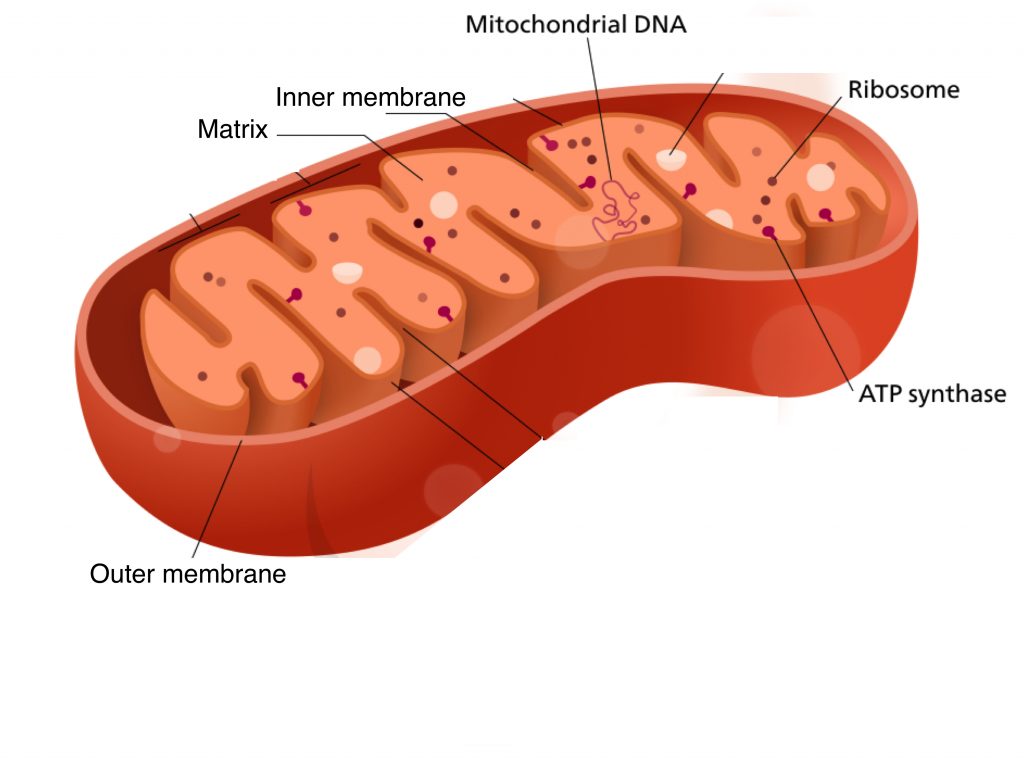
The oxidation of substrates takes place in mitochondria, and this provides the energy for oxidative phosphorylation, which also happens there. The key intermediates linking the two parts of energy metabolism are the redox carriers, which name is a shortened version of reduction-oxidation carriers. These molecules have aromatic ring structures, which allows them to be reversibly reduced and oxidized by accepting, or giving up, electrons. Substrate oxidation provides electrons that reduce redox carriers in mitochondria. The electrons are then transferred down a chain of other redox carriers, eventually to oxygen, to form water. As the redox carriers pass on their electrons they become oxidized again, and are used to re-oxidize more substrate.
The crux of oxidative phosphorylation is, how the energy released upon re-oxidation of redox carriers is used to drive ATP synthesis. None of the mechanisms proposed previously stood up to experimental probing. Peter Mitchell proposed instead that the energy released upon the re-oxidation of reduced redox carriers was used to pump protons, H+ ions, across the inner mitochondrial membrane. This produced an electrochemical (proton) gradient, and the membrane became an energy storage device, like a battery (5). Next, he proposed, there was molecular machinery that would use this energy to drive the synthesis of ATP. The overall process would be a reversal of ATP-dependent ion transport across a membrane against a concentration gradient.
This time, experimental evidence backed up the crazy scheme. Clever approaches were used to impose proton gradients across the inner membrane in isolated mitochondria — and this caused the mitochondria to synthesize ATP. (Actually, the experiment was first carried out with spinach chloroplasts, which generate ATP in a similar way (6).) Mitchell the iconoclast had rewritten the textbooks. In 1978, Peter Mitchell’s brilliant, unconventional theory won for him a Nobel Prize for Chemistry (7).
The other shoe drops
Mitchell didn’t discover the molecular machine that uses the energy of the proton gradient to synthesize ATP from ADP. But other people were already on the case, and a protein complex was isolated from the inner mitochondrial membrane that converted ATP to ADP and Pi. It was first identified as an “ATPase”, which hydrolyzes ATP. But on closer inspection it was found to link Mitchell’s proton gradient to ATP synthesis, because of its ability to catalyze the opposite reaction, ATP synthesis (all biochemical reactions are, at least in theory, reversible). This ATP synthase, as it was re-named, had the properties needed.
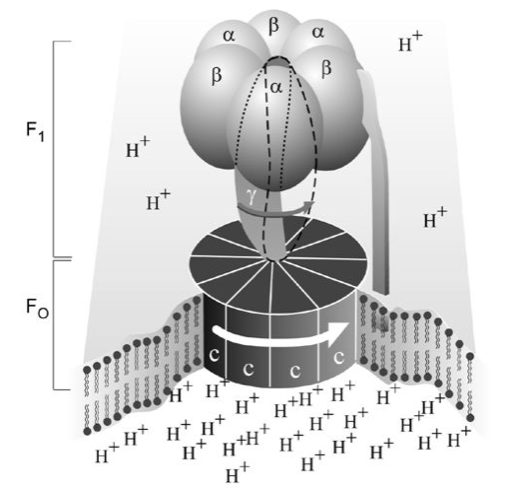
Embedded in the inner mitochondrial membrane, the ATP synthase is like a revolving door of a hotel; protons can pass through only by causing it to rotate. And this rotation forces ADP into juxtaposition with Pi, which generates ATP. Nineteen years after Peter Mitchell won his Nobel Prize, the discovery of the ATP synthase, too, led to a Nobel Prize for its discoverers, Paul Boyer and John Walker, which they shared with Jens Skou for his earlier discovery of ATP-driven ion transport of sodium and potassium across cellular membranes (8). Everything is connected to everything else, as the naturalist John Muir once said.
Some bacteria can swim by rotating a propeller, called a flagellum, that sticks out of one end. In some bacteria, the flagellar assembly contains components that are evolutionarily related to subunits of the mitochondrial ATP synthase (9). This is of course consistent with the idea that mitochondria began as captured ancient bacteria.
Coupling
Now we come to the connection between oxidative phosphorylation and the death of the body builder (or weightlifter — the report doesn’t say, but DNP is favoured by both groups) from DNP toxicity in Minneapolis. Normally, the protons that form a gradient across the inner mitochondrial membrane can re-enter the mitochondrial matrix only by passing through the ATP synthase complex. And this can happen only if the ATP synthase has a supply of ADP; i.e. only if ATP is being broken down to ADP through cellular energy-requiring processes. In turn, the reduced redox carriers cannot be re-oxidized until they pump protons across the inner mitochondrial membrane; the two processes are coupled. When the proton gradient builds up, it eventually stops further proton transport; you can only charge a battery so much. So oxidative metabolism itself grinds to a halt. Substrates, from stored or recently digested food, are oxidized only as rapidly as energy is needed. Everything is connected to everything else.
That is, until an uncoupling agent such as DNP is added. DNP inserts itself into the inner mitochondrial membrane by virtue of its chemistry, and there it provides a channel (DNP is called an “ionophore”) for protons to leak directly into the mitochondrial matrix, without going through the ATP synthase. It’s an effective way to lose weight — food is being oxidized, without regard for the body’s need for energy. Only heat is produced. The result is similar to what happens if the poles of a battery are connected to each other with a copper wire; the battery discharges precipitously and heat is produced. When the young man in Minneapolis ingested DNP, it inserted itself into the inner mitochondrial membranes of his cells; he experienced a raging, uncontrollable fever, and his muscles seized up from loss of ATP. Death soon followed.
Uncoupling also has a benign face. Babies have a volume-to-surface area problem: because of their small dimensions, the ratio of surface area to volume is large, and they lose heat fast. Nature helps out by providing them with brown fat, fat rich in mitochondria that are bathed in a protein called “Uncoupler 1” (several uncoupler proteins are known). This uncouples mitochondrial proton gradients just a little, and generates more heat than regular, adult, compulsively coupled mitochondria. And baby stays warm.
Quotation: I could not but be impressed by the great divergence of outlook, and even mutual antagonism, between the students of membranes and transport on the one hand, and the students of metabolic enzymology on the other hand – and I soon determined to try to understand both points of view in the hope that they might be brought together. (P. D. Mitchell, Nobel lecture)
Go to Latest Posts
Sources
- McGillis, E., A. Arens, T. Olivers, T. Gray, S. Blanchard, S. A. Love, C. L. Haynes, et al. 2018. Rapid-onset Hyperthermia and hypercapnea preceding rigor mortis and cardiopulmonary arrest in a CNP overdose. North American Congress of Clinical Toxicology Abstracts.
- Grundlingh, J., P. I. Dargan, M. El-Zanfaly, and D. M. Wood. 2011. 2,4-dinitrophenol (DNP): a weight loss agent with significant acute toxicity and risk of death. Journal of medical toxicology : official journal of the American College of Medical Toxicology 7:205-212.
- Mahase, E. 2019. Fat burning supplements: doctors warned to look out for toxicity after six deaths. BMJ 365:l2251.
- Robson, A. M., J. M. Kissane, N. H. Elvick, and L. Pundavela. 1969. Pentachlorophenol poisoning in a nursery for newborn infants. I. Clinical features and treatment. The Journal of pediatrics 75:309-316.
- Mitchell, P. 1961. Coupling of Phosphorylation to Electron and Hydrogen Transfer by a Chemi-Osmotic type of Mechanism. Nature 191:144-148.
- Jagendorf, A. T., and E. Uribe. 1966. ATP formation caused by acid-base transition of spinach chloroplasts. Proceedings of the National Academy of Sciences of the United States of America 55:170-177.
- Mitchell, P. 1991. Foundations of vectorial metabolism and osmochemistry. Bioscience reports 11:297-344; discussion 345-296.
- Boyer, P. D., B. Chance, L. Ernster, P. Mitchell, E. Racker, and E. C. Slater. 1977. Oxidative phosphorylatioin and photophosphorylation. Ann. Rev. Biochem. 46:955-1026.
- Imada, K., T. Minamino, A. Tahara, and K. Namba. 2007. Structural similarity between the flagellar type III ATPase FliI and F<sub>1</sub>-ATPase subunits. Proceedings of the National Academy of Sciences 104:485.