One of the most tantalizing aspects of cancer biology is this: that the normal immune system often recognizes growing tumors, and even targets them for destruction, but then is silenced. This story is about how to wake it up and mobilize it to greater anti-tumor activity. It is a story that reaches back a hundred years, to the innovative work of an esteemed German biochemist. It was his conviction that he had identified a key feature of cancer that would provide an opening for its treatment. He was right in his identification of such a feature, and it appears he was quite likely right about it providing an opening for cancer therapy. But it has taken almost until the present time to identify the basis of his observations, and it’s an ongoing challenge to apply that finding to therapy. This is about that history, and describes a new, potentially exciting, development stemming from it.
The Molecules of Silence
Tumor cells undergo changes that allow them to evade normal defensive tactics, either of the immune system, or anti-cancer drugs. An example of the first type is the occurrence of molecules on cancer cells that ‘turn off’ the attack of tumor-seeking lymphocytes, the so-called ‘Killer T Cells’ (lymphocytes of the T cell lineage, one of several types of lymphocytes).
The T cells circulating through the human body can recognize millions of molecular shapes, or antigens. Some are molecules like bacterial toxins that are presented to the T cells on ‘professional antigen-presenting cells’. (A certain amount of jargon is necessary; there won’t be much more.) Other antigens occur on the surfaces of cancer cells. T cells have a vast repertoire of sensing molecules, the receptors for antigens, and individual items from that repertoire can be amplified when a need is identified by the system.
The example below illustrates one mechanism by which a tumor can evade destruction by Killer T cells. In this case, a T lymphocyte (blue) has found a tumor cell (magenta) and will engage it and begin its destruction. However, the tumor cell has evolved a defensive mechanism, in the form of a molecule it displays on its outer membrane called PD-L1 (for ‘Programmed Death – Ligand 1’). This molecule has a shape and charge that causes it to bind tightly to a molecule called PD1, which is normally present on the surface of T lymphocytes. When engaged by PD-L1, PD1 delivers a ‘stand-down’ signal to its T cell.
This PD-L1 – PD1 interaction is part of the normal immune system; it prevents the autoimmune destruction of normal cells and tissues. But because the cancer cell in this example produces PD-L1 in sufficient numbers, it signals the killer T lymphocyte to not engage, to stay quiescent and float harmlessly away. And the cancer cell will then go on growing and replicating.
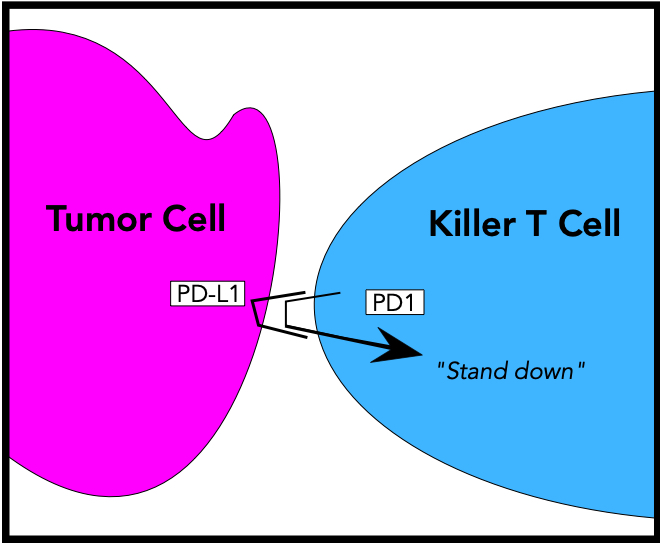
In a tussle that resembles the spy-vs-spy struggle of Mad Magazine fame, medical scientists have developed tools to neutralize the PD-L1 defensive weapon of cancer cells. One of these anti-defense weapons is an antibody, manufactured by genetic engineering, that recognizes and covers up the PD1 molecules displayed by T cells. Kaytruda is the trade name of one such drug. Kaytruda (and other agents that work by such a mechanism, which are called ‘checkpoint inhibitors’) recognizes PD1 and binds to it, blocking its interaction with PD-L1 on cancer cells. The killing of tumor cells ensues. And if you’re wondering whether Kaytruda can lead to autoimmune attack because of this, yes it can. It’s a potential side-effect, which can be serious. Efficacy depends on using carefully calibrated amounts of Kaytruda.
There’s always a need for new tools in the spy trade, and a different approach has recently been developed to stop the inactivation of Killer T Cells. It introduces into those cells a molecule that activates them, and which signals that something needs to be done; a criminal element has been engaged. The beauty of this approach is that an intrinsic property of tumor cells is used to focus that activating molecule to where it’s needed, namely, adjacent to the tumor. The story behind that has almost a 100-year history. It began with the work of a famous German biochemist named Otto Heinrich Warburg.
A Privileged and Talented Young Man
If you wanted to draw a stereotypical picture of a privileged, young, upper-class German male of the early twentieth century you could do worse than studying Otto Warburg. He was born in 1883 into a family of prominent bankers, scholars, and artists. His father Emil occupied the Physics Chair at the University of Berlin, considered to be the most prestigious position of its kind in Germany. Emil was also a fine pianist, who often played chamber music with his accomplished amateur violinist friend Albert Einstein. The Nobel Prize winners Emil Fischer (for chemistry) and Max Planck (physics) were frequent visitors to the Warburg home. Other leading physicists, chemists, and physiologists were family friends.
Surrounded by all of this towering scientific talent and achievement, it’s not surprising that young Otto had ambition; he confessed that it was his goal to be a greater scientist than his father. By the time he was 28, he had completed a doctorate in Chemistry (with Fischer) and an M. D. degree.
World War I started when Otto was 31, and, as a patriotic German, he enrolled in an elite horse regiment. (He was a skilled equestrian.) Military service resulted in both a serious wound and an Iron Cross, First Class. Einstein then intervened to get Warburg out of harm’s way because he perceived him to have brilliant scientific potential; Warburg went along, because by this time, 1918, he thought that the war was hopeless for Germany.
Warburg was dedicated to scientific research. Really dedicated. He would begin lab work at 8:00 AM, following an hour of horse riding, which was almost his only distraction from research. The workday ended for his technicians only when Warburg went home, which was usually after supper had been taken in the laboratory. For Warburg, this was followed by more work.
Warburg never married. From 1918 until his death in 1970, a fellow cavalry man named Jakob Heiss lived with him. Warburg led a charmed existence during the Nazi era; Hitler was supposedly so paranoid about cancer that he kept close his most famous cancer researcher, the homosexual Jew Warburg.
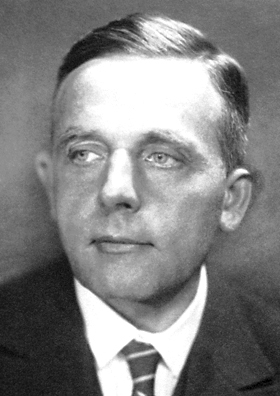
Warburg’s bearing was erect, and although he wasn’t tall, he had a domineering way of interacting with people, who tended to be overpowered by the intense gaze of his pale blue eyes. He almost never admitted to being wrong, even when he was shown by other people to be so. He hated administration, teaching, and being bored. (He shared the latter feeling with J. D. Watson, the co-father of the double helix, who advised “Avoid boring people.” Presumably Jim Watson used ‘boring’ as an adjective, not a verb.)
The Surprising Cancer Cell
Warburg demonstrated a high scientific capability as a young man. By age 25, he had published an important paper on the rate of oxygen consumption by sea urchin eggs, showing that upon fertilization, it went up six-fold. His explanation was that the fertilized egg became highly activated, and needed more energy to carry out its functions of growth and production of the embryo, and this would require more oxygen (he was right).
Warburg’s interest in the rates and chemical mechanisms of cellular energy production (for which he won a Nobel Prize in 1931) led him to study the energy metabolism of cancer cells. He had devised laboratory equipment that made it easier to measure the oxygen consumption rates of tissue slices and cells. He was aided in this by his connections to the physics community, many members of which were friends of his family. (This instrument, the ‘Warburg apparatus’, was still being used in the laboratory where I began my Ph. D. studies in the early 1960s.)
Warburg turned to the study of cancer cells. He thought that, like fertilized sea urchin embryos, rapidly growing cancer cells would have an increased oxygen consumption, compared to normal cells. But what he found was exactly opposite: cancer cells consumed less oxygen than normal ones, even though they had a higher rate of fuel (carbohydrate) consumption. (Although loath to admit error, he did yield to his own experimental data.) The cancer cells were using mainly ‘anaerobic’ (oxygen-independent) metabolism. He found this difference in all of the cancer cells he studied in the laboratory. Although cancer cells did use oxygen, they had a much higher ratio of anaerobic to aerobic energy metabolism than normal cells.
When Warburg further found that if he deprived certain kinds of cells of oxygen they became tumors, he concluded that damage to aerobic metabolism was the cause of cancer, which was a conclusion too many. Having made a brilliant experimental insight, he tried to interpret and apply his result where it wasn’t warranted. In fact, cancer cells have perfectly serviceable oxidative metabolism. He continued to promote the idea that impairment of oxidative energy metabolism was a primary cause of cancer, and even in his 70s he rejected the growing evidence that cancer is a disease of the genes.
But his finding that cancer cells rely more heavily on anaerobic energy metabolism than normal cells in the presence of oxygen is correct. It has recently led to a novel approach to cancer therapy.
What’s Really Going On?
Today we have an overall understanding of what’s going on in the energy metabolism of cancer cells (although the fundamental basis for their preference for anaerobic energy metabolism is not yet fully understood). The difference between typical cancer cells and normal ones is similar to the difference between a short-course sprinter and a marathon runner.
The sprinter relies almost completely on energy metabolism that is oxygen-independent (that is, it is anaerobic). Although oxygen is important, it isn’t much involved in the ten seconds or so of extreme exertion that a 100-meter sprinter engages in. She’s relying mainly on a kind of metabolism called glycolysis, in which sugars are converted to lactic acid without the participation of oxygen.
Glycolysis produces acid, both as its end product lactic acid, and by the mechanism of the metabolic pathway itself. This acid needs to be neutralized by oxygen-dependent metabolism, and it is, as the runner, doubled over with hands on thighs, gasps for air during post-race recovery.
A marathon runner, on the other hand, continually depends on oxygen for energy production; if his rate of energy expenditure exceeds the capacity of his muscles to use oxygen, he runs out of energy and has to stop running. And this aerobic metabolism uses up the materials that, in the sprinter, would go into lactic acid.
Warburg’s discovery that cancer cells, like the sprinter, are heavily dependent on oxygen-independent energy metabolism even in the presence of oxygen meant that they produce acid. Recently it was found that cancer cells pump that acid out and create an acidic microenvironment. That excited some cancer researchers because it offered a potential target for therapy.
Why drugs work
Drugs that stop infections (antibiotics) or the growth of cancer almost always rely for their effectiveness on the fact that they target aspects of bacterial, or viral, or tumor cell growth that are different from normal cells. For example, penicillin, the first wonder drug, interferes with cell wall synthesis of bacteria, and stops their growth. It’s an aspect of bacterial growth (at least of gram-positive bacteria) that animal cells don’t share; they don’t have cell walls of this type at all.
Many anticancer drugs target cellular properties that are at least to some degree shared with normal cells — after all, cancer cells are ‘normal’ cells growing out of control — but may stop the growth of particularly fast-growing cells, and thus have some specificity. This targeting of normal components or processes makes antitumor drugs difficult to use — there’s sometimes a narrow window of therapeutic efficacy between a useful anticancer effect and toxicity to normal tissue.
The excitement generated by the discovery of the acidic microenvironment of tumors, which is due to the effect first described by Otto Warburg nearly 100 years ago, is that this might provide a target. This has recently prompted a group of investigators to try to increase the ability of the immune system to target tumors in their acidic microenvironment.
These researchers, who are at Yale University and the University of Rhode Island, knew that the reason the immune system couldn’t eliminate many tumors was that the tumors ‘camouflaged’ themselves, or, as researchers say, they were ‘immunologically cold’ — the immune cells didn’t see them as anything unusual, or at least unusual enough, to get stirred up. The PD1 — PD-L1 interactionj is one such silencing mechanism.
The researchers already knew that the immune system could be engaged if certain agents were used to co-stimulate them. These agents entered the T cells and turned on genes necessary for cellular activation. The problem was, that to be useful, these activating agents needed to be focused on getting into immune cells adjacent to the tumor, not ones distributed throughout the body; the latter could cause autoimmune reactions. Their idea was to use the acidic environment of the tumor to focus the immune-stimulating molecules onto passing T lymphocytes.
The strategy used by these researchers was to create a new class of agents, which consisted of one molecular entity (a protein) that preferentially inserts into cell membranes in an acidic microenvironment, and one molecular entity that would stimulate immune cells (the latter is an analog of a normal intracellular activating signal). The two components of the hybrid construct had to separate once inside the T cell. That was achieved by linking them with a bond that is automatically cleaved under intracellular conditions.
Did it work? As far as it has been tested, yes. Mice bearing human tumors that normally kill them were ‘cured’. Moreover, this effect depended on T lymphocytes, so the mechanism was probably as imagined. Even better, mice cured of the tumor were then immune to re-inoculation of the same tumor. But of course, only mice were being saved. Whether it works in humans is the subject of the next steps of investigation. This work is described in user-friendly form in an on-line article.